Lithium halides are attractive as conversion cathodes for potentially high energy-density lithium batteries but have not been explored deeply in aprotic electrolytes.
In this issue of Joule, Wang, and co-workers describe a set of design principles of the electrode and electrolyte to enable the reversibility of lithium halide/graphite mixture cathodes for lithium batteries. By establishing a general rule of liquefaction of the (inter)halogen and rationally designing the electrolyte, they introduced the interhalogen-graphite intercalation compounds as a new family of conversion cathodes for high-energy rechargeable batteries.
As the widespread use of Li-ion batteries (LIBs) continues to increase, significant concerns regarding the cost and resource availability of transition metal oxides as conventional cathodes are apparent. In particular, cobalt and nickel reserves are facing scarcities that will lead to considerable increases in the cost of cathode materials in the near future. Moreover, these transition metal oxide cathodes (LiMO2, M = Co, Ni, Mn, etc.) have nearly approached their theoretical capacity limits and are becoming the limiting factor for further increasing the energy density of LIBs for a longer driving range. To overcome these shortcomings, immense research efforts have been directed toward transition-metal-free conversion cathodes, such as sulfur and O2, which are both abundant and have high theoretical capacity values. Unfortunately, these conversion cathodes face their own challenges, holding them back from commercial applications.
Another set of attractive conversion cathodes are the halogen/halide redox couples, which exhibit high capacity values at high potentials (e.g., 4.0 V for LiCl, 3.5 V for LiBr, and 3.0 V for LiI) and do not likely suffer from the resource scarcity. Iodine has been explored as a cathode material for Li batteries. Unfortunately, chlorine-based electrodes that promise high specific capacity have rarely been investigated due to challenges surrounding the halogen reactivity with the electrolyte and the evolution of the gaseous charged product, i.e., Cl2. In this issue of Joule, Wang and co-workers address these challenges and demonstrate reversible lithium halide/graphite mixture cathodes for Li batteries. It is worth noting that this new progress on the cathode side can be coupled with any anode materials, e.g., graphite and lithium metal. The authors looked into liquefying the halogen species and designing a quasi-ionic liquid electrolyte which suppresses dissolution of the halogens and allows for the formation of a robust cathode electrolyte interphase (CEI).
A number of strategies to overcome the loss of gaseous halogen-charged products have been attempted recently. In 2019, Wang’s group demonstrated a halogen conversion-intercalation cathode chemistry of a mixture electrode of graphite with LiCl and LiBr, whereby intercalation of an interhalogen of BrCl molecules into a graphite host occurs with the simultaneous departing of Li-ions to a graphite anode in a highly concentrated “water-in-bisalt” electrolyte. The halogen conversion chemistry benefits from the higher boiling point of the interhalogen species (e.g., 5°C for BrCl), as compared to the pure Cl2 (i.e., −34°C). In their new work, Wang et al. established a generic liquefaction approach for forming stable (inter)halogen-intercalated graphite compounds during charging.
At room temperature, Cl2 cannot be intercalated into the galleries of graphite, whereas at −30°C, they observed reversible redox behaviors of Cl2/Cl− with the graphite electrode. In addition, when the LiCl-graphite cathode is mixed with another lithium halide salt containing a less-electronegative halogen (i.e., LiBr or LiI), the electrode converts the gaseous Cl2 into BrCl or ICl interhalogen species of higher boiling points, which can then be reversibly (de)intercalated into graphite (Figure 1A). Through these approaches, the authors consider that liquefaction is a prerequisite to accessing the reversibility of the (inter)halogen cathodes.
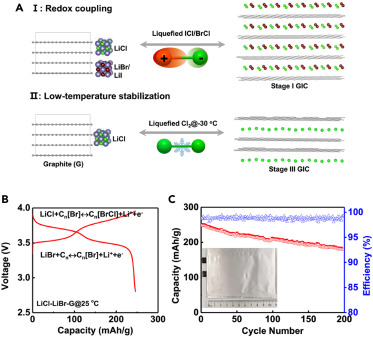
Figure 1 Generic rule of liquefaction and the design of a quasi-ionic liquid electrolyte enables the reversibility of lithium halide cathodes.
These design principles of electrode and electrolyte reveal the potential of using lithium halides as alternative transition-metal-free cathodes. In particular, the LiCl-LiBr-graphite cathode shows considerable promise with a high operation potential of 3.7 V, a high reversible capacity of 250 mAh/g based on the mass of both the lithium salts and graphite (Figure 1B), and an initial CE of 91.9%. Impressively, a LiCl-LiBr-graphite||Li (20 μm in thickness) pouch cell with a cathode loading of 2.5 mAh/cm2 can achieve 200 cycles with an average CE of 99.5% at 0.3C (Figure 1C). The demonstrated high specific energy of 770 Wh/kg (based on the total mass of both electrodes) highlights the potential impacts of these design principles of lithium halide cathodes in Li batteries.
Of note, the lessons learned from these design principles may not be specific only to lithium halides. The research herein shows how salt composite cathodes comprising a salt and an anion host structure can be applied in nonaqueous battery systems. These salt composite electrodes represent an uncharted research trajectory, which has only recently begun to be explored. Such electrode systems offer a potential solution for different battery systems, including dual-ion batteries. Enabling these new cathodes may also allow us to break through the current energy density ceiling of LIBs, which is imposed by the capacity limits of the transition metal oxide cathodes being used today.
Original Article: Reversible Halogen Cathodes for High Energy Lithium Batteries