Electrolyte design and interphases
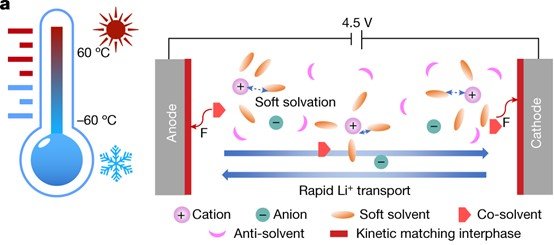
State-of-the-art electrolytes based on carbonate esters fail to meet most of the requirements for extreme lithium (Li)-ion batteries (LIBs) because their voltage window is limited to 4.3 V, they have a narrow operating temperature range of −20 °C to +50 °C and they are highly flammable.
To enhance the electrochemical performance, rational electrolyte design and regulated interfacial chemistry are crucial for high energy/power batteries that utilize high-capacity lithium metal/silicon anodes coupled with high-voltage cathodes.
Our group aims to develop electrolyte design strategies, ranging from aqueous electrolytes to nonaqueous electrolytes, and to solid electrolytes. Reveal the correlation between the solvation structure of the electrolyte and SEI/CEI interphases via in-depth study and push the scientific frontiers of next-generation high-energy batteries.
Advanced characterizations
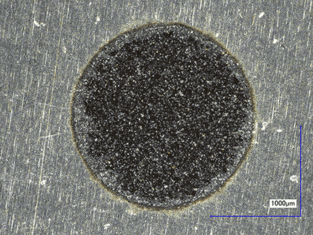
It is extremely challenging to fully understand the SEI/CEI formation mechanism due to the complicated SEI/CEI compositions which are still not completely identified so far.
Inspired by a number of meaningful explorations have been developed in recent years, we get to know the mysterious SEI/CEI better. SEI and CEI interphases are dynamic during the charge/discharge cycling, and therefore, advanced operando characterizations are crucial to understanding the interphase evolution. We try to guide the optimization of better electrolytes based on observations from various characterizations.
Anionic redox-based cathodes
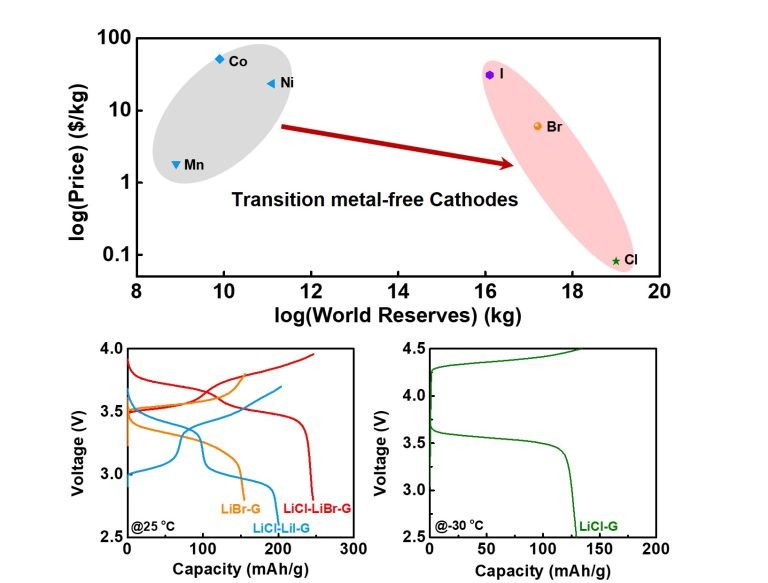
The energy density of Lithium-ion battery technology is mainly limited by transition metal oxides LiMO2 (M = Ni, Mn, or Co) cathodes, which are based on intercalation chemistry with modest capacity (< 200 mAh/g) and restricted charge voltage (< 4.3 V). Besides, conventional LiMO2 cathodes contain large quantities of cobalt and nickel also increases the raw material costs due to rapidly increasing prices for cobalt ($76,000/ton) and nickel($18,000/ton). It is crucial to explore new battery chemistries with transition metal-free cathodes.
Our lab has reported a series of lithium halide cathodes based on halogen conversion. These anion conversions can deliver an energy density of >850 Wh/kg, higher than all the LiMO2 and Li-rich transition metal oxide cathodes.
Our goal is to fundamentally understand the anionic redox chemistry via synchrotron X-ray absorption, electron-loss spectroscopy and 3D chemical/morphological imaging. Optimization of high-voltage and high-capacity anionic redox-based cathodes and compatible electrolytes to enable full cells to achieve >400 Wh/kg and cycle life >500 at cell level.